Whereas the intrinsic aging is believed to occur similarly in different ethnic groups [2], there are well-known ethnic differences in extrinsic skin aging [102–104]. They show higher rates of these processes in Caucasians than in Asians [103,105], which was generally connected with different melanin content and composition in these ethnic groups [2]. For example, the middle-aged Caucasian women demonstrate a much higher appearance of wrinkles as the Asian women from the same age group [103]. Severe wrinkles on the upper lip were found in 38% and 10% of French and Japanese women aged between 50 and 64 years, respectively. Some types of wrinkles were shown to appear approximately 15 years earlier in French than in Japanese women [103]. Similar differences were observed between German and Japanese women [106]. If dermal adipocytes are involved in the skin aging processes, these differences should be at least partly connected with ethnic variations in dWAT content.
Irradiation of human adipose-derived stem cells (ADSCs) with UVA in vitro demonstrated suppression of adipogenic differentiation potential of these cells. Such suppression could be observed already by very low fluence of 0.05 J/cm2 and was gradually more severe as fluence increased up to 5.0 J/cm2 [41]. This effect was connected with an observed significant down-regulation of PPARγ expression caused by UVA and demonstrated strong dose- dependent effects. Accumulation of triglycerides in UVA-irradiated cells in this study was also significantly reduced in a dose-dependent manner. Taking into account that UVA of such low fluence as 0.05 J/cm2 was able to induce the pronounced effect on the ADSCs, we can assume that a direct effect of UVR on dermal adipocytes is possible, since such fluences can easily be realized in vivo in the lower dermis. Later, the same group has shown that UVA in similar doses also modulates the “stemness” of ADSCs [42].
These results demonstrate that adipocytes can react even to low doses of UVR with a suppression of PPARγ expression and adipogenic differentiation as well as with a reduced accumulation of triglycerides in mature adipocytes. This additionally supports the idea that not only systemic pathways, but also direct local responses in dWAT, can be involved in the reaction of sWAT to UVR in vivo, as observed in [37].
Ergothioneine, a sulfur-containing micromolecular histidine derivative, has attracted increasing attention from scholars since it was confirmed in the human body. In the human body, ergothioneine is transported and accumulated specifically through OCTN-1, especially in the mitochondria and nucleus, suggesting that it can target damaged cells and tissues as an antioxidant. It shows excellent antioxidant, anti-inflammatory effects, and anti-aging properties, and inhibits melanin production. It is a mega antioxidant that may participate in the antioxidant network system and promote the reducing glutathione regeneration cycle. This review summarizes studies on the antioxidant effects of ergothioneine on various free radicals in vitro to date and systematically introduces its biological activities and potential mechanisms, mostly in dermatology. Additionally, the application of ergothioneine in cosmetics is briefly summarized. Lastly, we propose some problems that require solutions to understand the mechanism of action of ergothioneine. We believe that ergothioneine has good prospects in the food and cosmetics industries, and can thus meet some needs of the health and beauty industry.
Damage to this skin barrier function can lead to skin diseases or infections, such as atopic dermatitis (AD) and acne. Rizzo et al. [100] demonstrated that the p53 family p63 might be key in driving AD. Moreover, p53 affects cell proliferation and differentiation by regulating RUNX1, which is generally detected in the nucleus of epidermal cells but is not significantly expressed in patients with AD [101]. This may suggest that it is related to the reconstruction of the skin structure and the repair of the skin barrier.
Interestingly, OCTN-1 expression was strongly correlated (score = 0.999) with RUNX1 gene expression, reflected in the STITCH database, as shown in Figure 5. Moreover, a study has demonstrated that EGT has a positive effect on immune system diseases caused by abnormal RUNX1 expression such as rheumatoid arthritis (RA) [102,103,104] and non-alcoholic fatty liver disease [12]. Additionally, RUNX1 expression—similar to that of p63 and p53—can directly regulate the process of cell proliferation and differentiation and is also a vital regulatory factor for normal hematopoiesis in the body, which may be consistent with the result that the blood contains high concentrations of EGT [101]. Moreover, it is a key regulator of normal hematopoiesis in the body [105], which may be consistent with the abundant accumulation of EGT in blood [15]. Furthermore, the regulatory effect of RUNX1 on SLC22A4 and the activation effect of ROS on p53 may suggest that EGT is transported to clear excess ROS through the high expression of OCTN-1 or that the anti-inflammatory effect of inhibiting IL-33 alleviates AD after the skin barrier function is impaired. This may lead to the downregulation of the IL-33-mediated inflammatory markers (loricrin, keratin 1, and keratin 10) [106]. Overall, these findings suggest that EGT may play a significant role in remodeling skin barrier function and treating AD.
Figure 4. EGT potentially regulates RUNX1 via the protective skin barrier. Additionally, EGT can potentially downregulate the inflammatory factor IL-33 induced by MAPK kinase, reduce the production of inflammatory markers (loricrin, keratin 1, and keratin 10), and indirectly regulate apoptosis caused by p53/63 by inhibiting the excessive increase in ROS. Interestingly, EGT and RUNX1 protein expression induce a strong binding energy related to the proliferation and differentiation of keratinocytes or skin stem cells, and ultimately promote the renewal of skin barrier function.
Figure 5. Prediction of EGT binding with related proteins by STITCH database cluster analysis.
We document CDKis expression in vivo during hair follicle stem cell (HFSC) homeostasis and find p21 (cyclin-dependent kinase inhibitor 1a, Cdkn1a), p57, and p15 up-regulated at quiescence onset. p21 appears important for HFSC timely onset of quiescence. Conversely, we find that Runx1 (runt related transcription factor 1), which is known for promoting HFSC proliferation, represses p21, p27, p57, and p15 transcription in HFSC in vivo. Intriguingly, in cell culture, tumors, and normal homeostasis, Runx1 and p21 interplay modulates proliferation in opposing directions under the different conditions.
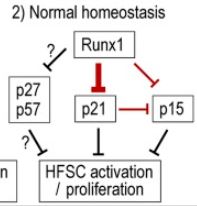